H2 Gas Reduces Neurologic Injury in Circulatory ArrestScientific Research
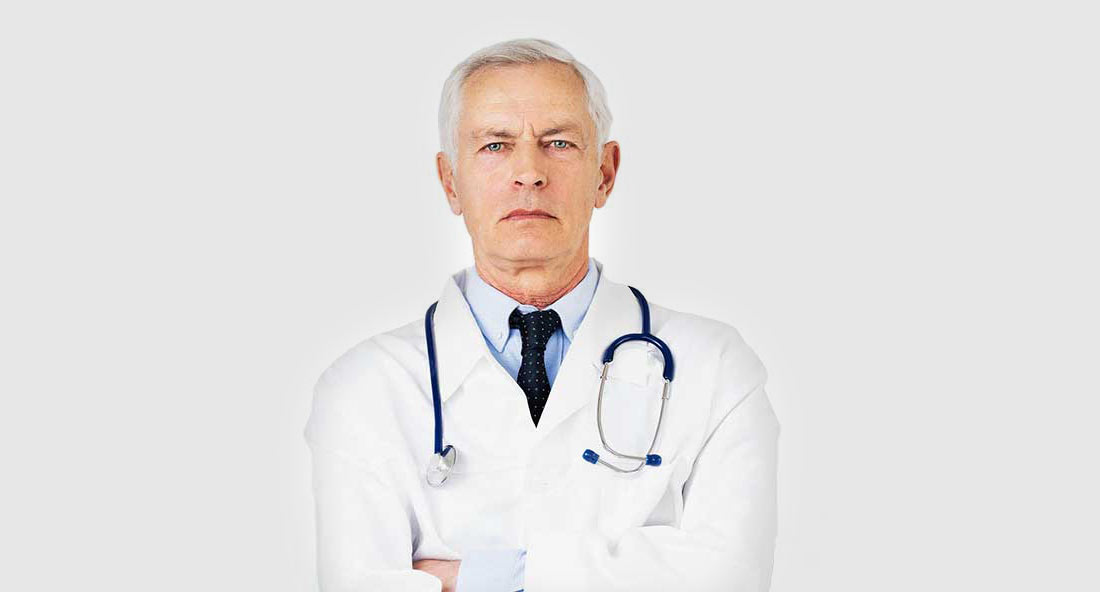
Perioperatively Inhaled Hydrogen Gas Diminishes Neurologic Injury Following Experimental Circulatory Arrest in Swine
Alexis R. Cole
aDepartment of Cardiology, Boston Children’s Hospital, Boston, Massachusetts
Dorothy A. Perry
aDepartment of Cardiology, Boston Children’s Hospital, Boston, Massachusetts
bDepartment of Pediatrics, Harvard Medical School, Boston, Massachusetts
Ali Raza
aDepartment of Cardiology, Boston Children’s Hospital, Boston, Massachusetts
bDepartment of Pediatrics, Harvard Medical School, Boston, Massachusetts
Arthur P. Nedder
cAnimal Resources at Children’s Hospital, Boston Children’s Hospital, Boston, Massachusetts
Elizabeth Pollack
cAnimal Resources at Children’s Hospital, Boston Children’s Hospital, Boston, Massachusetts
William L. Regan
dDepartment of Cardiovascular Surgery, Boston Children’s Hospital, Boston, Massachusetts
Sarah J. van den Bosch
aDepartment of Cardiology, Boston Children’s Hospital, Boston, Massachusetts
Brian D. Polizzotti
aDepartment of Cardiology, Boston Children’s Hospital, Boston, Massachusetts
bDepartment of Pediatrics, Harvard Medical School, Boston, Massachusetts
Edward Yang
eDepartment of Radiology, Boston Children’s Hospital, Boston, Massachusetts
fDepartment of Radiology, Harvard Medical School, Boston, Massachusetts
Daniel Davila
gDepartment of Neurology, Boston Children’s Hospital, Boston, Massachusetts
hDepartment of Neurology, Harvard Medical School, Boston, Massachusetts
Onur Afacan
eDepartment of Radiology, Boston Children’s Hospital, Boston, Massachusetts
fDepartment of Radiology, Harvard Medical School, Boston, Massachusetts
Simon K. Warfield
eDepartment of Radiology, Boston Children’s Hospital, Boston, Massachusetts
fDepartment of Radiology, Harvard Medical School, Boston, Massachusetts
Yangming Ou
bDepartment of Pediatrics, Harvard Medical School, Boston, Massachusetts
eDepartment of Radiology, Boston Children’s Hospital, Boston, Massachusetts
fDepartment of Radiology, Harvard Medical School, Boston, Massachusetts
Brenda Sefton
dDepartment of Cardiovascular Surgery, Boston Children’s Hospital, Boston, Massachusetts
Allen D. Everett
iDivision of Pediatric Cardiology, Johns Hopkins University School of Medicine, Baltimore, Maryland
Jeffrey J. Neil
eDepartment of Radiology, Boston Children’s Hospital, Boston, Massachusetts
fDepartment of Radiology, Harvard Medical School, Boston, Massachusetts
Hart G.W. Lidov
hDepartment of Neurology, Harvard Medical School, Boston, Massachusetts
jDepartment of Pathology, Boston Children’s Hospital, Boston, Massachusetts
kDepartment of Pathology, Harvard Medical School, Boston, Massachusetts
John E. Mayer
dDepartment of Cardiovascular Surgery, Boston Children’s Hospital, Boston, Massachusetts
lDepartment of Surgery, Harvard Medical School, Boston, Massachusetts
John N. Kheir
aDepartment of Cardiology, Boston Children’s Hospital, Boston, Massachusetts
bDepartment of Pediatrics, Harvard Medical School, Boston, Massachusetts
Associated Data
- Supplementary Materials
- Supplemental Figures S1–S8 and Supplemental Table 1mmc1.pdf (1.2M)GUID: AD5D5007-E593-48DF-AD21-A1D7D8AA53C8
Visual Abstract
Highlights
- •Inhaled hydrogen gas has been shown to temper the sequelae of ischemic insults. Its application in cardiopulmonary bypass has not been investigated.
- •Neonatal swine were cannulated to cardiopulmonary bypass and exposed to prolonged circulatory arrest (75 min at 25°C). Swine were randomized to treatment with or without inhaled 2.4% hydrogen gas mixtures for 24 h during and following ischemic injury. Hydrogen-treated swine exhibited significantly less severe brain injury than controls, as quantified by clinical examination, serology, magnetic resonance-graded volume of injury, and histopathology. Hydrogen treatment also decreased renal injury.
- •The administration of inhaled 2.4% hydrogen gas mixtures through a standard ventilator and anesthesia machine were safe, even in the setting of electrocautery.
Summary
This study used a swine model of mildly hypothermic prolonged circulatory arrest and found that the addition of 2.4% inhaled hydrogen gas to inspiratory gases during and after the ischemic insult significantly decreased neurologic and renal injury compared with controls. With proper precautions, inhalational hydrogen may be administered safely through conventional ventilators and may represent a complementary therapy that can be easily incorporated into current workflows. In the future, inhaled hydrogen may diminish the sequelae of ischemia that occurs in congenital heart surgery, cardiac arrest, extracorporeal life-support events, acute myocardial infarction, stroke, and organ transplantation.
Newborns with critical congenital heart disease often undergo major surgical interventions in the neonatal period that require the use of cardiopulmonary bypass (CPB). Several studies have provided radiographic evidence showing that new ischemic injury occurs following CPB 1, 2, 3, 4, 5, 6, 7. Neonates with the diagnosis of left heart obstructive lesions are consistently at the highest risk of cerebral injury 5, 8. In 1 study (1), new white matter injury (i.e., not present preoperatively) was evident in more than 70% of neonates undergoing aortic arch reconstruction. Cerebral injuries included moderate or severe white matter injury in 40% to 50% of patients; new infarctions were found in one-third of patients. Further, clinically evident seizures have been reported in up to 20% of neonates following surgery for congenital heart disease and are more common in patients undergoing prolonged deep hypothermic circulatory arrest 9, 10. Subclinical seizures occur in an even higher fraction 1, 10, 11. The presence of postoperative seizures is an important marker of underlying ischemic injury, which may manifest as radiologic injury and developmental delay years later (12). Thus, although abnormal neurodevelopment in infants with critical congenital heart disease is multifactorial (including in utero, genetic, and socioeconomic risk factors) (13), injury occurring during CPB represents a significant contributor to neurologic impairment.
To mitigate this problem, nearly all operations are performed under some degree of hypothermia, which suppresses cerebral oxygen consumption (14), and enhances preservation of high-energy phosphates, and reduces the accumulation of toxic metabolites (15). Cerebral hypoxia can be monitored using cerebral near-infrared spectroscopy and the degree and duration of cerebral hypoxia have been associated with subsequent neurologic impairment. For example, newborns experiencing a regional cerebral oxyhemoglobin saturation index <40 exhibited worse receptive communication at 2 years of age than those who did not (16). Efforts to minimize cerebral hypoxia during congenital heart surgery have resulted in improvements in neurologic outcomes. For example, the addition of carbon dioxide during hypothermia (i.e., pH-stat, which promotes cerebral vasodilation during bypass) was associated with a more rapid return of normal electroencephalographic activity (17). In another study (18), target hematocrit during CPB was significantly associated with Psychomotor Development Index (PDI) scores at 1 year of age (18).
At a cellular level, cerebral hypoxia during CPB creates a complex cascade of changes within the inner mitochondrial membrane, causing formation of the superoxide anion radical (O2−•) (19), which in turn generates hydroxyl radicals (•OH) by the Fenton reaction. The •OH is the strongest of the oxidant species and reacts indiscriminately with nucleic acids, lipids, and proteins, causing direct cellular injury and stimulating apoptosis. Because there is no known detoxification system for •OH, scavenging •OH is a critical antioxidant process (20). Recently, it has been discovered that hydrogen gas (i.e., molecular dihydrogen [H2]) selectively reduces •OH in vivo (Figure 1) (21). For example, rodents breathing either 2% or 4% H2 for 90 min following a period of middle cerebral artery occlusion exhibited a substantially smaller infarct volume, improved neurologic scores, weight gain, and thermoregulation relative to controls (21), findings that were repeated by an independent group (22). In another study (23), H2-treated rodents undergoing a 5-min period of asphyxial cardiac arrest exhibited more favorable neurologic scores, improved myocardial function, and improved 96-h survival than did those treated with targeted temperature management alone. Here, we studied the effects of inhalational H2 gas on neurologic outcomes in neonatal swine undergoing cerebral hypoxic-ischemic injury in the setting of hypothermic CPB. We hypothesized that the inhalation of H2 gas surrounding a CPB-related ischemic injury would diminish the degree of neurologic injury in subject animals relative to that in control animals.
Presumed Mechanism of H2 Action in the Setting of Ischemia
Ischemic insults create tissue hypoxia, stimulating a complex cascade (not shown) that results in the release of superoxide (O2−•). When O2−• is present in excess (i.e., when compensatory mechanisms become saturated), it directly causes the reduction of transition metal ions, including Fe3+ and Cu2+, which in turn, generates hydroxyl radicals (•OH) by the Fenton reaction. The •OH is the strongest of the oxidant species and is the direct effector of DNA injury and lipid membrane peroxidation, which releases HNE and MDA, causing direct cellular injury and stimulating apoptosis. Unlike O2−• and H2O2, there is no known detoxification system for •OH; therefore, scavenging •OH is a critical antioxidant process. Molecular hydrogen (H2), which freely permeates the cell wall and diffuses into the cytosol and mitochondria, reduces the hydroxyl radical to water and thus mitigates •OH-mediated tissue injury. HNE = 4-hydroxyl-2-nonenal; MDA = malondialdehyde; SOD = superoxide dismutase.
Methods
The following protocol was approved by the Institutional Animal Care and Use Committee at Boston Children’s Hospital (protocol 15-08-2990) (Figure 2), which included a review of hydrogen-related environmental hazard concerns.
Study Protocol
Neonatal swine were acclimated with bottle feedings 5 time per day (blue marks) for 5 days prior to experimentation. On the day of experimentation, swine were anesthetized and instrumented for cardiopulmonary bypass. Ischemic injury included circulatory arrest for 75 min at 25°C. Swine were then rewarmed and decannulated and underwent mechanical ventilation using a standardized intensive care protocol. Swine were ventilated for a total of 24 h (including pre- and post-operative treatments) with or without 2.4% inhaled hydrogen therapy (n = 8 swine per group). Videotaped neurologic examinations (green marks) took place prior to and daily following the circulatory arrest period. Swine underwent sedated brain MRI followed by terminal cerebral perfusion for histopathologic examination on postoperative day 3. F = Friday; M = Monday; MRI = magnetic resonance imaging; R = Thursday; RN = nursing care; S = Saturday/Sunday; W = Wednesday.
Experimental protocol
Sixteen neonatal female Yorkshire swine (3.8 to 5.8 kg; post-natal age: 6 to 10 days of life) were acclimated to their surroundings and bottle fed 5 times daily by research staff for 6 days. On the day of experimentation, swine were anesthetized by intramuscular injections of tiletamine (Telazol), xylazine and atropine, and tracheally intubated. Swine were then sedated by using inhaled isoflurane (0.25% to 2%). Neuromuscular blockade (cisatracurium) was administered upon anesthetic induction and then again prior to sternotomy incision. A right femoral arterial (3-F sheath) catheter and a right internal jugular venous (4-F, 5 cm) catheter were placed and continuously transduced. Esophageal and rectal temperature probes were placed. A median sternotomy was performed, a subtotal thymectomy performed, and the pericardium opened. A sterile human infant-sized CPB circuit (S5 infant perfusion pack, Sorin Group, Arvada, Colorado) was primed with blood from an adult donor swine sacrificed on the previous day. The right atrium and ascending aorta were cannulated (18-F DLP malleable single-stage venous and 10-F arterial cannulas, Medtronic-Biomedicus, Eden Prairie, Minnesota), and full-flow CPB was instituted. As is our institution’s clinical practice, a dose of methylprednisolone (30 mg/kg intravenous [IV]) was administered upon initiation of CPB. Swine were then cooled to 25°C (measured rectally) over 30 min, using a pH-stat management strategy (carbon dioxide added). Following cooling, the aorta was cross-clamped, and a solution of cold blood, potassium, magnesium, and lidocaine were administered into the aortic root, and cardioplegia was induced (del Nido Cardioplegia Solution [24]), causing prompt diastolic arrest. Circulation was then discontinued for a 75-min period of circulatory arrest. Rectal temperature was maintained as close to 25°C as possible by using surface cooling as needed. Following circulatory arrest, circulation was restored, and swine were warmed to 37°C over 60 min. Swine were then weaned from CPB using inotropic support as needed to maintain systolic blood pressure >80 mm Hg. Cannulas were removed, hemostasis ensured, and the sternum closed.
Survival period
Sedation was then transitioned to infusions of propofol (1 to 3 mg/kg/h) and fentanyl (1 to 2 μg/kg/h), and inhalational isoflurane was discontinued for an 18-h period of regimented intensive care staffed by intensive care nursing staff. During this time, esophageal temperature was continuously monitored and maintained below 38°C by using a cooling blanket. Blood pressure was maintained with an infusion of dopamine (3 to 5 μg/kg/min, titrated to systolic blood pressure >70 mm Hg). Diuresis was achieved by using furosemide (1 mg/kg every 12 h). Mechanical ventilation was continued by using synchronized, intermittent mandatory ventilation with a fraction of inspired oxygen, required to maintain pulse oximetry saturation of 95% and target tidal volumes of 8 ml/kg. Animals were continuously monitored for clinical seizure activity. Seizures lasting longer than 2 min were treated according to a protocol of lorazepam (0.1 mg/kg IV every 5 min up to 3 doses), then phenobarbital (20 mg/kg IV every 20 min for 2 doses), then fosphenytoin (20 mg/kg IV every 20 min for 2 doses), then an increase in the rate of propofol infusion (up to 10 mg/kg/h). Inotrope score was calculated as: [dopamine (μg/kg/min) + dobutamine (μg/kg/min) + 100× epinephrine (μg/kg/min)] (25).
After the animals underwent 18 h of intensive care, the arterial catheter, thoracic drain, and tracheal tube were removed. Swine were then observed for 3 days, with quantification of neurologic status by daily neurologic examinations. Blood drawn prior to and daily after the injury was analyzed for complete blood count, chemistry profile, hepatic transaminases, and venous blood gas analysis. Glial fibrillatory acidic protein (GFAP) was assessed using an electrochemiluminescent sandwich immunoassay (Meso Scale Diagnostics, Rockville, Maryland), with a detection range of 0.001 to 40.0 ng/ml (26).
Inhaled hydrogen therapy
Animals were randomized to treatments as described above with or without inhaled hydrogen (2.40%) for a 24-h period during and after the ischemic injury (n = 8 per group). At the beginning of the study, we created a table that dictated the treatment allocation for each experiment in random order, according to which patients were treated. Due to environmental hazards and logistical considerations, members of the veterinary, perfusion, and overnight nursing staff were not blinded to treatment group allocation, whereas surgical staff, neurologists, and histopathologists were blinded to treatment allocation. Premixed, certified hydrogen gas blends containing 2.40 ± 0.05% of grade-6 purity (99.9999%) hydrogen gas with either balance medical air or medical oxygen (Praxair Distribution, Inc., Jessup, Maryland) were obtained and received as nonflammable gas mixtures. These tanks were fitted with a 50-psi regulator and a flash arrestor and then attached directly to the air and oxygen (respectively) inlets of the anesthesia machine (Dräger Apollo, Coppell, Texas) during the experimental period and to the mechanical ventilator (Servo I model, Maquet, Gothenburg, Sweden) during the survival period (Supplemental Figure S1). Additional hydrogenated “carbogen” mixtures were made, including 0%, 4%, 6%, and 8% carbon dioxide, 2.40% hydrogen, and balance oxygen for use during hypothermic perfusion. Ambient hydrogen concentrations were measured continuously (Eagle 2 model, RKI Instruments, Union City, California).
Swine neurologic deficit scores
Swine were evaluated prior to and following each 24-h period after the injury using a previously described swine neurologic deficit score (SNDS) (27) by 2 research technicians, present for each examination (unblinded to treatment allocation), and 2 neurologists (by review of videotaped examinations, blinded to treatment allocation). The mean of the 4 scores was taken at each time point. The examination assessed cranial nerve function, respiratory pattern, motor and sensory function, level of consciousness, and behavior, each assigned a total of 100 points. Points were assigned based on specific abnormal neurologic findings (Supplemental Table 1), such that a score of 0 was normal, and a score of 500 represented brain death. The presence or absence of clinical seizures was not part of the scoring system.
Brain magnetic resonance imaging
On day 3 post-injury, swine were anesthetized for brain magnetic resonance imaging (MRI) (3-T Skyra model scanner, 64-channel head and neck coil, Siemens, Corp., Munich, Germany). High-resolution T1, T2, fluid-attenuated inversion recovery, and diffusion-weighted images were obtained. Areas of enhancement on axial and coronal T2 and apparent diffusion coefficient images were manually assessed on a voxel-per-voxel basis and outlined (itk-SNAP software application, Penn Image Computing and Science Laboratory, University of Pennsylvania, Philadelphia, Pennsylvania, and Scientific Computing and Imaging Institute, University of Utah, Salt Lake City, Utah) by a radiology technician (Mr. Abdelhakim Ouaalam, Department of Radiology, Boston Children’s Hospital, Boston, Massachusetts), a radiologist (E.Y.), and a clinical neurologist (J.N.), all of whom were blinded to treatment allocation. From these values, total volumes of cranial injuries per swine were calculated using in-house software normalized to brain volume, and the total volumes of injuries were compared between groups by using the Mann-Whitney U test.
Neurohistopathology
Following brain MRI of the swine on post-injury day 3, both carotid arteries and jugular veins were then cannulated by cutdown and perfused with normal saline (2 l), followed by 4% paraformaldehyde (4 l). The heads of swine were fixed in 10% formaldehyde for 24 h and then removed and paraffin embedded and then stained for hematoxylin and eosin. Hypoxic-ischemic injuries of the frontal cortex, temporal cortex, hippocampus, dentate gyrus, caudate nucleus, and thalamus were graded according to a previously defined scale by a pathologist (H.G.W.L.) blinded to treatment allocation. Briefly, histologic injury was evaluated by using both light and fluorescence microscopy (28) on a scale of 0 through 5 for each of 6 regions, as follows: 0 = normal, no injury; 1 = rare hypereosinophilic neurons; 2 = small clusters of hypereosinophilic neurons; 3 = majority of neurons (>50%) are hypereosinophilic; 4 = significant damage to neurons; and 5 = cavitated infarction with histologic necrosis. Animals that did not survive for 3 days (due to refractory status epilepticus) were assigned the median histologic score for animals in the control group.
Statistical analysis
The primary outcome of this study was neurologically intact survival, which was defined as an SNDS of ≤120 at 3 days, compared between groups using a log-rank test (Gehan-Breslow-Wilcoxon test). On the basis of a previous series of pilot experiments, 15% of animals in the control group were expected to meet this endpoint, and the study was powered to identify the fact that 70% or more in the hydrogen treatment group met this endpoint at 5 days with 80% power and an alpha level of 0.05.
Between-group differences in SNDS, regional neurohistologic scores, body temperatures, regional oxyhemoglobin saturation index values, hemodynamics, inotrope scores, serum lactic acid concentrations, blood gas concentrations, PaO2/FiO2 ratios, chemistry values, and hematologic parameters were assessed over time by using 2-way repeated measures analysis of variance (ANOVA), using Prism version 7.00 software (for MacIntosh [Cupertino, California], GraphPad, La Jolla, California). In order to complete ANOVA, missing values for the 2 animals which were sacrificed early were estimated to be the median values across controls for that time point. When results were statistically significant, time-dependent differences between groups were assessed by using Sidak’s multiple comparisons test. Interobserver reliability for SNDS was assessed by Pearson coefficient between blinded versus unblinded observers for time-matched pairs. Single time point values, such as differences in cerebral infarct volumes or changes in GFAP relative to baseline, were compared between groups by Student’s t-test or Mann-Whitney U test, as appropriate. For all tests, a p value of <0.05 was considered statistically significant.
Results
Hydrogen hazards
Hydrogen-oxygen and hydrogen-air mixtures were administered via the anesthesia machine and mechanical ventilator without malfunction or incident. Electrocautery was used with no subjective difference in performance between controls and the hydrogen-treated animals. Measurements of ambient hydrogen concentrations were below the lower limit of detection (<1 ppm) at all time points.
Clinical outcomes
All animals were successfully weaned from CPB. The degrees to which hypothermia was achieved were similar between the groups (mean rectal temperature: 27.4 ± 1.8°C vs. 26.5 ± 1.9°C in controls and hydrogen-treated groups, respectively; p = 0.2387) (Supplemental Figure S2). Cerebral and somatic near infrared spectroscopy values were also similar between groups, frequently reaching a nadir of <20 during the deep hypothermic circulatory arrest period (Supplemental Figure S3). Two swine in the control group exhibited refractory status epilepticus and were sacrificed at 32 and 36 h post-injury following a failed trial of extubation; no hydrogen-treated animals exhibited seizures. Survival to 3 days was similar between groups (log rank test: p = 0.1435). Hydrogen-treated swine exhibited a higher rate of neurologically intact survival, defined as an SNDS of ≤120 at the time of death (log-rank test; p = 0.0035) (Figure 3A). SNDSs were significantly improved in hydrogen-treated swine in the postoperative period (p < 0.0001) (Figure 3B). Interobserver reliability was excellent among in-person scorers (i.e., unblinded research team members) and videotaped reviewers (i.e., blinded neurologists) (Pearson correlation coefficient: 0.895). The increase in serum GFAP concentrations relative to those at baseline was significantly higher in controls than in H2-treated swine at 60 min post-injury (p = 0.0068) (Supplemental Figure S4).
Clinical Neurologic Outcomes
(A) Hydrogen-treated swine exhibited significantly higher rates of neurologically intact survival, which was defined as an SNDS ≤120 at the time of death (log-rank test: p = 0.0035). The overall rates of survival were similar between groups (log-rank test: p = 0.1435). (B) Hydrogen-treated swine exhibited significantly lower SNDS at 1, 2, and 3 days post injury (2-way ANOVA: p < 0.0001). Data for day 1 represent 8 swine per group; data for days 2 and 3 represent 8 swine in the hydrogen group and 6 in the control group (2 swine allocated to the control group died from refractory seizures and could not be successfully extubated). ***p < 0.001; **p < 0.01 for daily differences according to Sidak’s multiple comparisons post test. ANOVA = analysis of variance; DHCA = deep hypothermic circulatory arrest; SNDS = Swine Neurodevelopment Score.
Relative to controls, H2-treated swine exhibited a significantly lower level of serum creatinine during the survival period (p = 0.0152), an average of 0.38 mg/dl lower by postoperative day 3. There were no differences in serum markers of hepatic injury or function (Supplemental Figure S5). There were no significant differences in acute hemodynamics, and inotrope scores were similar between the groups (Supplemental Figure S6). In the postoperative period, there were no differences in PaO2/FiO2 ratios as a marker of lung function (p = 0.92), nor were there significant differences in blood gas values during the postoperative period (Supplemental Figure S7). Similarly, there were no significant differences in hematologic endpoints between groups during the survival period (Supplemental Figure S8).
Neuroradiology
Swine in both groups exhibited a radiographic predominance of frontal and temporal lobe injuries. However, H2-treated swine exhibited significantly lower volumes of white matter injury on T2 imaging than did controls (median: 134 mm3 [interquartile range [IQR]: 84 to 200 mm3] in H2-treated swine vs. 383 mm3 [IQR: 77 to 639 mm3] in controls; p = 0.0460) (Figure 4).
Radiographic Differences Between Groups
Axial T2 images (A) were assessed for radiographically apparent injuries (B), which were outlined as moderate (green) or severe (red) on a voxel-per-voxel basis. These areas of injury were corroborated by review of apparent diffusion coefficient mapping (C), which were similarly outlined (D). (E) Areas of injury were rendered in 3 dimensions and overlaid onto an image of the brain to provide a visual image of the differences in the volume of cranial injury. Data were based on brain MRI of 8 swine in the hydrogen-treated group and 6 in the control group (2 swine allocated to the control group died from refractory seizures and could not survive to day 3). (F) H2-treated animals exhibited a significantly lower volume of injury than did control animals (Student t-test: p = 0.0463). The line represents median, boxes are interquartile ranges, and error bars are minimum and maximum values.
Neuropathology
Regions of radiographically apparent injury correlated well with histologically apparent injury, with a predominance of injury in the frontal cortex. As a group, H2-treated swine exhibited significantly lower histologic injury scores than did controls (p = 0.0044) (Figure 5), with a predominance of injury in the frontal cortex. There was no evidence of thalamic injury in this model.
Histopathologic Differences Between Groups
Regions of radiographically apparent injury (A) (arrows) correlated well with histologically apparent injury (B), shown here by fluorescence microscopy (original magnification: ×1, using a Rhodamine filter). Neuronal injury was scored between 0 (normal) and 5 (severe neuronal injury, necrosis) for each region through identification of hypereosinophilic and/or apoptotic neurons by using both light (C) and fluorescence (D) microscopy (original magnification: ×60; bars = 50 μm). Open arrows = hypereosinophilic and apoptotic neurons. (E) As a group, hydrogen-treated swine exhibited significantly lower histologic injury scores than controls (2-way repeated measures ANOVA according to Sidak’s test results: p = 0.0044). Data are based on histopathology for 8 swine in the hydrogen-treated group and 6 in the control group (2 swine allocated to the control group died from refractory seizures and did not survive to day 3). Data are means; error bars are SEM.
Discussion
We have shown that the perioperative administration of 2.40% H2 is safe and diminishes neurologic injury in an experimental model of circulatory arrest. Although the combination of temperature and duration of circulatory arrest used is not used clinically, the model did successfully establish the degree of neurologic injury manifested in the most severely affected neonates, including perioperative seizures and radiographically apparent injury. In that setting, the perioperative administration of H2 improved clinical neurologic scores, decreased serum markers of brain injury, decreased radiographically apparent volumes of brain injury, and lessened the degree of histopathologic injury. In addition, H2-treated swine exhibited a significantly lower concentration of serum creatinine during the survival period, suggesting that hydrogen may diminish the effects of renal ischemia. Notably, there were minimal differences between groups in injury measures of cardiac performance, such as venous oxyhemoglobin saturation. This may be because, in essence, animals underwent a 75-min period of cardioplegic aortic cross-clamping, an ischemic insult that is known to be well tolerated.
This work adds to a growing body of preclinical studies supporting the therapeutic efficacy of inhalational H2 gas. As mentioned previously, inhalational H2 gas has been shown to diminish the volume of brain injury in rodent models of middle cerebral artery occlusion (21) and asphyxial cardiac arrest (23). H2 inhalation has also been shown to decrease cellular injury and improve post-ischemic organ function in several animal models. For example, the administration of 1.3% H2 in dogs for 6 h following a 90-min occlusion of the left anterior descending artery resulted in a 50% reduction in infarct size (29). A similarly protective effect has been shown following experimental ischemia-reperfusion injury in liver (30), lung (31), heart (32), and small intestine (33) and in models of septic shock (34). Still other studies have examined the intravenous administration of H2-saturated saline (35) and the oral administration of H2 in tablet or water form (36), although the serum concentration achieved by the oral route is orders of magnitude lower than that in the inhalational route (37).
Recently, a series of bold first-in-human studies of inhalational H2 gas has been described. The first study (38) was a case series describing the administration of 2% H2 in 5 mechanically ventilated survivors of witnessed out-of-hospital cardiac arrest, which found that 4 of 5 patients exhibited favorable neurologic function (cerebral performance category 1) at hospital discharge. There were no environmental hazards reported. The second study (39) describes the face mask administration of 1.3% H2 in 10 adults (plus 10 controls) undergoing percutaneous coronary reperfusion for ST-segment elevation myocardial infarction, finding that H2 significantly improved ejection fraction at 6-month follow-up examination (39). A third study (40) described 25 patients (plus 25 controls) who presented with acute mild-to-moderately severe stroke, who underwent inhalation of 3% H2 gas through face masks for 1 h twice per day for 7 days, which resulted in significant improvements in U.S. National Institutes of Health Stroke Scale scores and volumes of cerebral infarction by diffusion-weighted MRI imaging (40).
The application of H2 administration in infants undergoing CPB is attractive for several reasons. First, it appears to be safe and easy to use. The dose tested here (2.4%) is a nonflammable gas mixture, even when mixed with balance (i.e., 97.6%) oxygen; hydrogen concentrations above 4% are known to be flammable. Following due diligence, we were able to attach these source gases were directly to the anesthesia machine and mechanical ventilator, and did not note any adverse effects on the delivery of anesthetic gas or on the function of either device. This represents an improvement on prior delivery techniques (which add a hydrogen-nitrogen mixture to inspiratory gas following passage through the ventilator) (38) in several ways. The setup described in the present study ensured delivery of a constant concentration of H2 regardless of the patient’s oxygen requirements. Administration of H2 gas mixtures to the ventilator inlet would likely be required to treat infants due to their high bias flow and rapid respiratory rate, factors that would cause excessive dilution of even the most concentrated hydrogen-nitrogen mixture. Second, H2 appears to be well tolerated at the doses tested. Consistent with prior reports 40, 41, we did not find that the administration of H2 had a measurable effect on hemodynamics or lung function. In the future, it will be important to study the effects of more extended durations of exposure (e.g., 72 h continuously) on these endpoints. Third, the application of H2 may be practically added to current therapies, including hypothermia. For these reasons, a clinical trial of perioperative H2 administration in neonates at high risk for neurologic injury may be warranted.
Study limitations
1) Although the newborn piglet has become an accepted model for the term neonate, we note that the maturity of myelination in these animals was approximately that of an 12-18 month old infant. 2) The protective effects of inhalational anesthetics and of intravenous sedatives (e.g. propofol) are well appreciated and may have affected the degree of neuronal injury, although the dosing was protocolized and equally applied to both groups. 3) Because we did not perform electroencephalography, we are unable to comment on hydrogen’s effect on subclinical seizure activity. 4) Although previously well-characterized, we did not quantify the arterial concentrations of hydrogen gas during administration. Based on prior work, we expect that the arterial concentration of 2.4% H2 (which was a certified gas mixture and therefore the concentration was independently verified) in mechanically ventilated swine would reach a plateau between 5-10 µM/l within 20 min of inhalation (41). The number of animals included in each group was small, such that less common adverse effects of hydrogen administration may not have been detected. A properly powered safety study is warranted.
Conclusions
In a small series of neonatal swine, the perioperative administration of inhalational H2 gas diminishes neurologic injury following experimental circulatory arrest.
Perspectives
COMPETENCY IN MEDICAL KNOWLEDGE: The use of inhaled hydrogen gas to diminish ischemic injury has been applied successfully in several rodent models and was recently described in humans following stroke, acute myocardial infarction, and cardiac arrest. A demonstration of safety in healthy volunteers is warranted, followed by a prospective study of hydrogen inhalation during congenital heart surgery and other clinical scenarios.
TRANSLATIONAL OUTLOOK: The favorable side effect profile and ease of administration make hydrogen a potentially appealing ancillary therapy.
Acknowledgments
The authors thank veterinary staff Cara Pimental, Madeleine Woomer, and Hugh Simonds; clinical perfusion staff Greg Matte, Kevin Connor, Natalie Toutenel, and Molly Bryant; overnight nursing staff Jay Hartford, Danielle Healey, and Stephanie Pietrafitta; clinical radiology staff Peter Morriss and Joseph Zmuda; and feeding volunteers Abigail Moore, Lindsay Thomson, Katherine Black, Andrew Lock, Jemima Lamothe, Yifeng Peng, Raymond Seekell, and Cameron Russell. The authors also thank Jie Zhu for performing GFAP assays; institutional safety officer Chad Pires, Boston Fire Department; and Centers for Disease Control and Prevention consultant Isaac Zlochower for assistance with the technical implementation of this work.
Footnotes
Dr. Kheir is supported by American Heart Association grant 15GRNT25700161; and by philanthropic donations from the Hess Family Cardiac Innovation Fund, the Furber Family Innovative Therapies Fund, and Lindsay Bartels (a donor who provided some financial support for the study). Dr. Everett is a consultant for Immunarray LLC; and holds patents through Johns Hopkins University assigned to Immunarray, Inc. All other authors have reported that they have no relationships relevant to the contents of this paper to disclose.
All authors attest they are in compliance with human studies committees and animal welfare regulations of the authors’ institutions and U.S. Food and Drug Administration guidelines, including patient consent where appropriate. For more information, visit the JACC: Basic to Translational Scienceauthor instructions page.
References
The Original Article:
original title: Perioperatively Inhaled Hydrogen Gas Diminishes Neurologic Injury Following Experimental Circulatory Arrest in Swine
Alexis R Cole, Dorothy A Perry, Ali Raza, Arthur P Nedder, Elizabeth Pollack, William L Regan, Sarah J Bosch, Brian D Polizzotti, Edward Yang, Daniel Davila, Onur Afacan, Simon K Warfield, Yangming Ou, Brenda Sefton, Allen D Everett, Jeffrey J Neil, Hart G Lidov, John E Mayer, John N Kheir
-
Abstract:
Highlights • Inhaled hydrogen gas has been shown to temper the sequelae of ischemic insults. Its application in cardiopulmonary bypass has not been investigated. • Neonatal swine were cannulated to cardiopulmonary bypass and exposed to prolonged circulatory arrest (75 min at 25°C). Swine were randomized to treatment with or without inhaled 2.4% hydrogen gas mixtures for 24 h during and following ischemic injury. Hydrogen-treated swine exhibited significantly less severe brain injury than controls, as quantified by clinical examination, serology, magnetic resonance-graded volume of injury, and histopathology. Hydrogen treatment also decreased renal injury. • The administration of inhaled 2.4% hydrogen gas mixtures through a standard ventilator and anesthesia machine were safe, even in the setting of electrocautery.